RLC Circuit Ohm's Law
This lecture combines the material of the previous two ones dedicated to the Ohm's Law in alternating current circuits.
The first one was analyzing a circuit with a resistor and a capacitor.
The second one analyzed a circuit with a resistor and an inductor.
This lecture analyzes a circuit with all three elements - resistor, inductor and capacitor.
Consider a closed AC circuit with a generator with no internal resistance producing a sinusoidal electromotive force (EMF or voltage)
E(t)=E0·sin(ωt)(volt)
where E0 is a peak EMF produced by an AC generator,
ω is angular speed of EMF oscillations and
t is time.
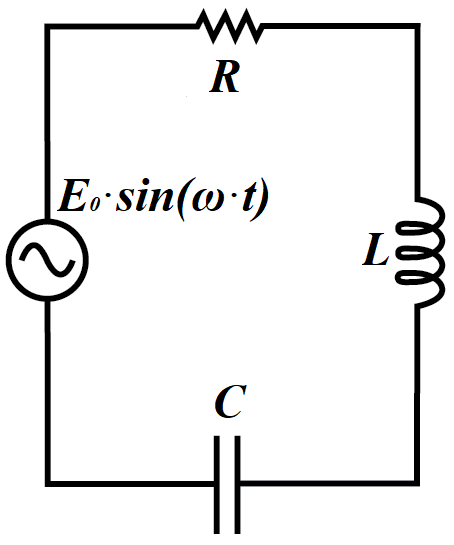
In this circuit we have a resistor with resistance R, an inductor with inductance L and a capacitor with capacitance C connected in series with EMF generator. This is RLC-circuit.
Since the circuit is closed, the electric current I(t) going through a circuit is the same for all components of a circuit.
Assume that the voltage drop on a resistor (caused by its resistance R) is VR(t), the voltage drop on an inductor (caused by its inductive reactance XL=ω·L) is VL(t) and the voltage drop on a capacitor (caused by its capacitive reactance XC=1/(ω·C)) is VL(t).
Since a resistor, an inductor and a capacitor are connected in a series, the sum of these voltage drops should be equal to a generated EMF E(t):
E(t) = VR(t) + VL(t) + VC(t)
As explained in the previous lectures the Ohm's Law localized around a resistor states that
I(t) = VR(t)/R or
VR(t) = I(t)·R
The inductance of an inductor L, voltage drop on this inductor caused by a self-induction effect VL(t), electromagnetic flux going through an inductor Φ(t) and electric current going through it I(t), as explained in the lecture "AC Inductors" of the chapter "Electromagnetism - Alternating Current Induction", are in a relationship
VL = dΦ/dt = L·dI(t)/dt
The capacity of a capacitor C, voltage drop on this capacitor VC(t) and electric charge accumulated on its plates QC(t), according to a definition of a capacitance C, are in a relationship
C = QC(t)/VC(t) or
VC(t) = QC(t)/C
We have expressed both voltage drops VR(t) and VL(t) in terms of an electric current I(t):
VR(t) = I(t)·R
VL(t) = L·I'(t)
Since an electric charge QC(t) is also involved to express the voltage drop on a capacitor, we will use this electric charge as a main variable, using the definition of an electric current as a rate of change of electric charge
I(t) = QC'(t) and
I'(t) = QC"(t)
Now all three voltage drops can be expressed as functions of QC(t) and, equating their sun to a generated EMF E(t), we can the following differential equation
E0·sin(ωt) = QC'(t)·R +
+ L·QC"(t) + QC(t)/C =
= L·QC"(t) + R·QC'(t) +
+ (1/C)·QC(t)
As we know, capacitive and inductive reactance are functionally equivalent to resistance, and they all have the same unit of measurement - ohm. Therefore, it's convenient, instead of capacitance C and inductance L, to use corresponding reactance XC=1/(ω·C) and XL=ω·L.
So, we will substitute
C = 1/(ω·XC)
L = XL/ω
Substitute QC(t)=y(t) for brevity. Then our equation looks like
(XL/ω)·y"(t) + R·y'(t) +
+ ω·XC·y(t) = E0·sin(ωt)
Solving this equation for y(t)=QC(t) and differentiating it by time t, we will obtain the expression for an electric current I(t) in this circuit as a function of all given parameters and time.
First of all, let's find a particular solution of this differential equation.
The form of the right side of this equation prompts to look for a solution in trigonometric form
y(t) = F·sin(ωt) + G·cos(ωt)
Then
y'(t) =
= ω·[F·cos(ωt)−G·sin(ωt)]
y"(t) =
= −ω²·[F·sin(ωt)+G·cos(ωt)] =
= −ω²·y(t)
Using this trigonometric representation of potential solution y(t), the left side of our equation is
(XL/ω)·y"(t) + R·y'(t) +
+ ω·XC·y(t) =
= −(XL/ω)·ω²·y(t) + R·y'(t) +
+ ω·XC·y(t) =
= −ω·XL·y(t) + R·y'(t) +
+ ω·XC·y(t) =
= R·y'(t) + ω·(XC−XL)·y(t) =
= R·ω·[F·cos(ωt)−G·sin(ωt)] +
+ ω·(XC−XL)·[F·sin(ωt)+
+G·cos(ωt)] =
= ω·[(XC−XL)·F−R·G]·sin(ωt)+
+ω·[R·F+(XC−XL)·G]·cos(ωt)
Since this is supposed to be equal to E0·sin(ωt), we have the following system of two linear equations with two variables F and G
ω·[(XC−XL)·F−R·G] = E0
ω·[R·F+(XC−XL)·G] = 0
or
(XC−XL)·F−R·G = E0/ω
R·F+(XC−XL)·G = 0
Determinant of the matrix that defines this system is
(XC−XL)²+R².
It's always positive and usually is denoted as
Z² = (XC−XL)²+R²
The value Z is called the impedance of a circuit and, as we will see, plays the role of a resistance for an entire RLC-circuit.
This system of two linear equations with two variables has a solution:
F = (E0/ω)·(XC−XL)/Z²
G = −(E0/ω)·R/Z²
Consider two expressions that participate in the above solutions F and G:
(XC−XL)/Z² and R/Z²
Since Z² = (XC−XL)²+R², we can always find an angle φ such that
(XC−XL)/Z = sin(φ) and
R/Z = cos(φ)
Using this, we express the solutions to the above system as
F = (E0/(ω·Z))·sin(φ)
G = −(E0/(ω·Z))·cos(φ)
Now the solution of the differential equation for y(t)=QC(t) that we were looking for in a format
y(t) = F·sin(ωt) + G·cos(ωt)
looks like
y(t)=(E0/(ω·Z))·sin(φ)sin(ωt) −
− (E0/(ω·Z))·cos(φ)cos(ωt) =
= −(E0/(ω·Z))·cos(ωt+φ)
As we noted before, differentiation of this function gives the electric current in the circuit I(t):
I(t) = y'(t) =
= (E0 /(ω·Z))·ω·sin(ωt+φ) =
= (E0 /Z)·sin(ωt+φ) =
= I0·sin(ωt+φ)
where I0=E0 /Z is an equivalent of the Ohm's Law for an RLC-circuit.
Similar relationship exists between effective voltage and effective current
Ieff = I0 /√2 =
= E0 /(√2·Z) = Eeff /Z
Here impedance Z, defined by resistance R, inductive reactance XL and capacitive reactance XC as
Z = √(XC−XL)²+R²
plays a role of a resistance in the RLC-circuit.
There is a phase shift φ of the electric current oscillations relative to EMF. It is also defined by the same characteristics of an RLC-circuit:
(XC−XL)/Z = sin(φ)
R/Z = cos(φ)
Hence
tan(φ) = (XC−XL)/R
This makes phase shift positive or negative depending on the values of capacitive and inductive reactance.
If XC is greater than XL, the phase shift is positive, if the reverse is true, the phase shift is negative.
If the values of reactance are the same, that is XC=XL, there is no phase shift. From definition of reactance, it happens when
1/(ω·C) = ω·L or
1/(L·C) = ω² or
L·C = 1/ω²
This relationship between inductance, capacitance and angular speed of EMF oscillation is call resonance.
Expressions for electric current
I(t) = (E0 /Z)·sin(ωt+φ) =
= I0·sin(ωt+φ)
in RLC-circuit, where impedance Z=√(XC−XL)²+R² and tan(φ)=(XC−XL)/R, correspond to analogous formulas for R-, RC- and RL-circuits presented in the previous lectures. All it takes is to set the appropriate value of capacitance or inductance to zero.
No comments:
Post a Comment