p-n Junction Diodes
Consider a semiconductor of n-type, like silicon (Si) with phosphorus (P) additive.
Atoms of P with its five valence electrons are embedded in the crystalline structure of Si with four of these valence electrons falling nicely into a structure, making covalent bonds with four neighboring atoms of Si, while the fifth one cannot get a pair to establish a covalent bond, so it's rather loosely attached to its atom of P and might randomly travel around.
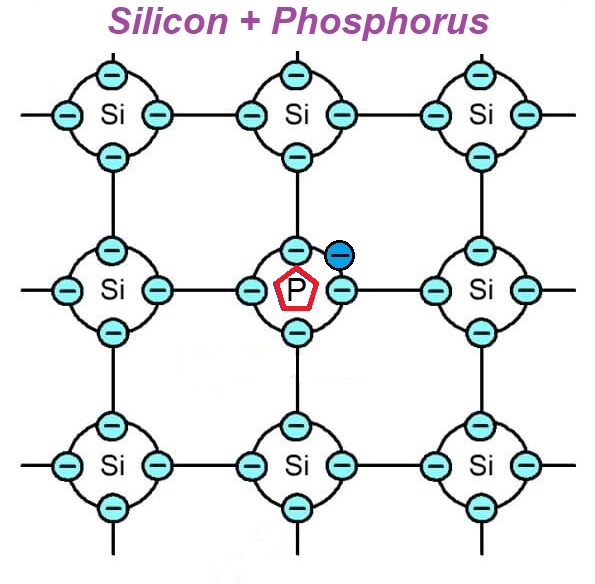
During this process of randomly fluctuating electrons within an n-type of a semiconductor the whole structure remains electrically neutral because these traveling negatively charged electrons are balanced by extra protons in atoms of phosphorus.
The number of these traveling electrons is not great, so, if we apply a low voltage to a semiconductor, we can observe some low conductivity, but increasing the voltage will show that the conductivity is greater. The mechanism of this conductivity is based on a combination of electrostatic forces and forces of covalent bonds that maintain a crystalline structure of a semiconductor.
Freely traveling excess electrons of an n-type semiconductor will be electrostatically attracted by a positive electrode that has deficiency of electrons. Then the body of a previously electrically neutral semiconductor becomes positively charged, since it lost some electrons. Consequently, electrons from a negative electrode will enter the semiconductor, will not be captured by covalent bonds and become free traveling ones, replacing those consumed by a positive electrode.
This process of electrons moving from a negative electrode through an n-type semiconductor to a positive electrode will continue, as long as the voltage between electrodes is maintained at sufficient level.
Now consider a p-type semiconductor, like silicon (Si) with boron (B) additive.
Atoms of B, embedded in the crystalline structure of Si, have their three valence electrons bonded to valence electrons of three surrounding atoms of Si, while one valence electron of the fourth neighboring atom of Si cannot get a pair to establish a covalent bond with atom of B, thus a hole in the crystalline structure is formed.
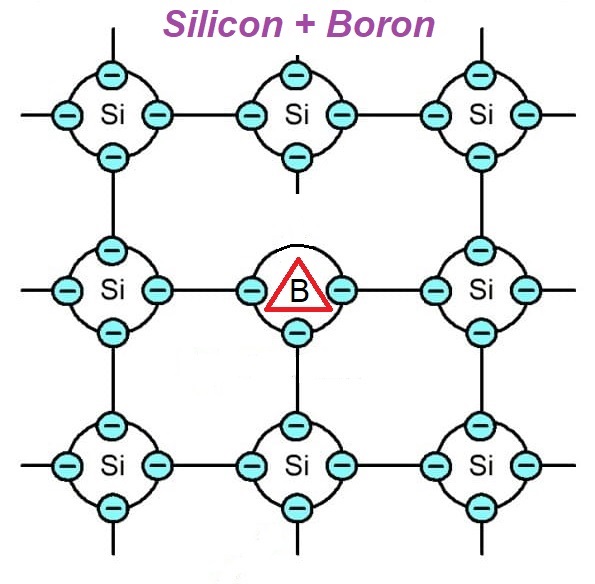
Any neighboring atom of Si, if properly excited, can have its valence electron to jump from its own atom to fill the gap. That leaves a hole in another spot of a crystalline structure. Then some other electron from yet another atom can fill the new hole, opening a hole in yet another area. So, holes in the crystalline structure are randomly traveling inside a p-type of semiconductor.
This process of randomly moving holes in the crystalline structure of p-type semiconductor is analogous to the above described random moving of electrons in the n-type semiconductor.
It also assures that, if a sufficient voltage is applied to this type of semiconductor, the conductivity can be observed. The mechanism of this conductivity is based on a combination of electrostatic forces and forces of covalent bonds that maintain a crystalline structure of a semiconductor.
Consider holes in the crystalline structure caused by a deficiency of one valence electron of atoms of B. There are electrons randomly traveling from a negative electrode into a semiconductor and back. Some electrons that happened to be in the vicinity of a hole will be captured by the forces of covalent bonds and fill the holes in the crystalline structure.
Then the semiconductor will become negatively charged, that is will have excess of electrons. The excess electrons will be attracted by a positive electrode and new holes will be created in the crystalline structure.
This process of electrons moving from a negative electrode through a p-type semiconductor to a positive electrode and, correspondingly, holes moving in the opposite direction will continue, as long as the voltage between electrodes is maintained at sufficient level.
Next topic is to analyze the behavior of electrons and holes at the border or junction between the n-type semiconductor and the p-type one. This border area is called p-n junction.
Initially electrically neutral, a semiconductor on the n-type side of a junction has electrons that do not fit in its crystalline structure and not involved in the covalent bonding.
On the p-type side of a junction, also initially electrically neutral, there are holes in the crystalline structure of a semiconductor waiting to capture some electron into a covalent bonding.
The first consideration is related to activity related to the forces of covalent bonds. Randomly traveling electrons from the n-type semiconductor can jump through a junction into the p-type semiconductor and be captured by covalent bonds to restore missing electrons of a crystalline structure of a semiconductor in the area adjacent to the junction.
That, in turn, creates a layer of negative charge near the junction on the p-type side and a positively charged layer near the junction on the n-type side.
Excess electrons kept by covalent bonds near the junction on the p-type side act as an electrostatic barrier to prevent new electrons from the n-type side to jump the junction.
Some kind of equilibrium is developed between electrons on the n-type side not involved in the crystalline structure and ready to jump the junction and electrons already jumped to the p-type side.
The covalent bonds are restored on the p-type side and there are no electrons not linked through covalent bonds on the n-type side of the junction. The price for this proper crystalline structure on both sides of a junction is that there is an excess electrons on the p-type side and deficiency of electrons on the n-type side.
Let's connect the p-type side of a junction to a positive electrode and the n-type to a negative electrode of a battery.
Excess electrons from the p-type will be attracted to a positive electrode and the barrier of electrons on the p-type side that prevented new electrons from the n-type to jump the junction will no longer be there.
This will cause new electrons from the n-type side to be able to jump the junction into p-type. In parallel, electrons from the negative electrode will be attracted to a positively charged n-type side, compensating electrons that have already jumped to the p-type side.
As we see, there will be an electric current through a p-n junction, as long as the voltage is maintained.
Now let's connect the electrodes in an opposite way. Positive to n-type side and negative to the p-type.
Electrons that do not fit a crystalline structure on the n-type will be attracted to a positive electrode and will no longer participate in the process.
New electrons from the negative electrode will penetrate the body of the p-type side filling all the covalent bonds and reinforcing the electrons' barrier near a junction to completely stop all flow of electrons from the n-type side.
Now the crystalline structure on both sides of a p-n junction are complete and no new electrons are traveling in any direction. There is no current through a p-n junction.
As we see, the electrons can go through a p-n junction only if n-type side is connected to a negative electrode and p-type side is connected to a positive electrode. So, p-n junction acts like a diode. This configuration of p-type and n-type semiconductors joined together and acting as a diode is called p-n junction diode.
No comments:
Post a Comment