Notes to a video lecture on http://www.unizor.com
Direct Current Motors
Direct current (DC) motors are used where the sources of electricity produce direct current, like batteries.
For example, DC motors work in car engine starter, computers, toys, drones and many other devices.
We will concentrate on principles of their work without going into many
details. Basically, we will describe these motors as they were first
thought of by their inventors. Obviously, initial ideas were further
improved by many people and improvements are still introduced after more
than 200 years after their invention, but the basic ideas are still
there.
Let's start with the idea of the DC motor.
The beginning of a development of a DC motor is associated with a simple
experiment we described before - the one that demonstrated the Lorentz
force on a current in a magnetic field.
If the direction of a current I is perpendicular to a direction of magnetic field lines B,
the magnetic field "pushes" the conductor that carries this current in a
direction perpendicular to both magnetic field lines and a current by
force F.
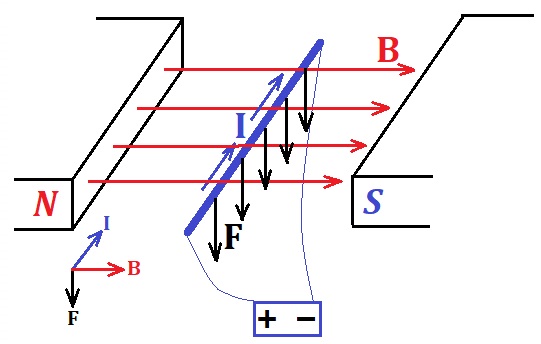
The first modification of this experiment was to use a rectangular wire
frame instead of a straight line conductor and let it spin around an
axis. The force exerted by a magnetic field will act on both sides of a
wire frame that are perpendicular to magnetic lines in opposite
directions, creating a rotational momentum, so the frame with rotate.
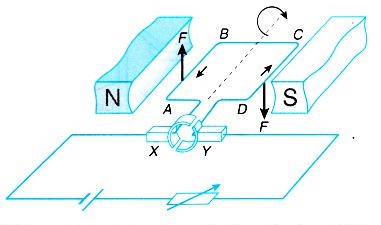
The force F of a magnetic field "pushes" segment AB up and segment CD, where the electric current goes in an opposite to segment AB direction, is "pushed" down by this force. This creates a rotational momentum.
The problem is, the rotation will stop when the wire frame plane will be
perpendicular to a direction of a magnetic field, because the forces
exerted by a magnetic field on both sides of a wire frame AB and CD
will no longer create a rotating momentum, they will act against each
other within the wire frame plane and after a short oscillation caused
by a rotational inertia the rotation stops.
On the picture above, when the plane of a wire loop reaches the position perpendicular to the magnetic field force, the segment AB will be on the top and the magnetic field force F will "push" this segment up. Segment CD will be on the bottom and the magnetic field force F
will "push" this segment down. Both forces are acting within the same
plane with the axis of rotation and nullify each other. No rotational
momentum is created.
Our next improvement is related to overcoming this problem.
What happens if exactly at the moment when our wire frame plane is
perpendicular to magnetic field, when the forces exerted by a magnetic
field no longer create a rotational moment, we switch off the electric
current in a wire and a very short moment later we switch it on, but in
the opposite direction of the electric current?
First of all, the rotation will continue by inertia during the time
electricity is off. Then, we turn the electric current on but in
opposite direction. That means, the direction of the magnetic field
force will change to an opposite. Segment AB will be "pushed" down, segment CD
will be "pushed" up. Since the wire frame has passed the point of
perpendicularity to magnetic field by rotational inertia during electric
current off time, the newly formed magnetic field forces will create a
rotational moment and the direction of rotation will be the same as
before.
Our frame will continue the rotation in the same direction until segment AB will be on the bottom and segment CD - on the top.
At that moment we will do the same switching off the electric current to
let our frame pass the perpendicular position towards the magnetic
field force, and a very short moment later we switch electric current on
in an opposite direction. Now segment AB will be "pushed" up again, segment CD will be "pushed" down, which will allow to complete the cycle of rotation and the rotation will continue in the same direction.
All we need is to explain how to switch the direction of an electric current.
Here is a drawing of this simple device called commutator.
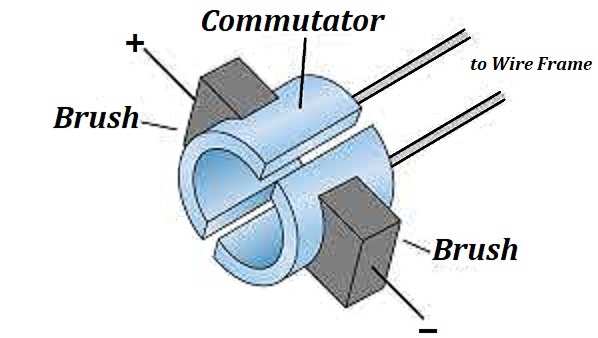
Direct current comes through brushes to a commutator and to a revolving
frame connected to it. As this construction revolves, the brushes lose
the contact with a commutator for a very short period, then again touch
the commutator, but with opposite poles.
That's how we change the direction of the current that forces the frame to constantly rotate in the same direction.
The inner rotating part of this type of a DC motor is called rotor, the outer stationary part with a magnet is called stator.
The detail implementation of this design of a DC motor is outside the scope of this course. Our purpose is to convey the idea.
The weak part of a DC motor design with brushes is that these brushes
wear out with time and are the source of sparks, which might be
prohibitive in some environments. Plus, they are noisy.
With development of electronics inventors came up with a better design
that does not involve brushes at all, rotation is accomplished without
any mechanical switches.
First step on the design of this brushless DC motor is to realize that
we have to invert the functionality of permanent magnet and a wire
frame. The need for a commutator with mechanical brushes was related to the fact that electric circuit was rotating.
Let's invert roles and put two parallel wire frames on opposite ends of a circle as a stator and a permanent magnet on an axis in the middle as a rotor.
Recall that a wire loop with direct current running through it acts like a magnet
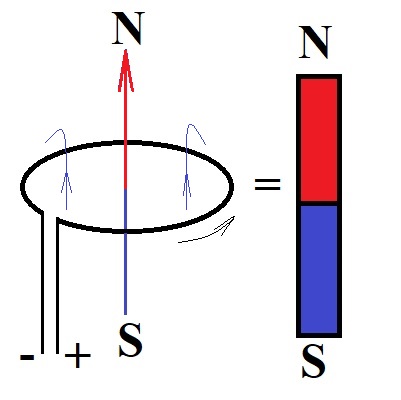
Putting two such loops on opposite ends of a stator creates a magnetic
field and a freely rotating permanent magnet in between will have to
turn to align itself with the field of these two wire loops
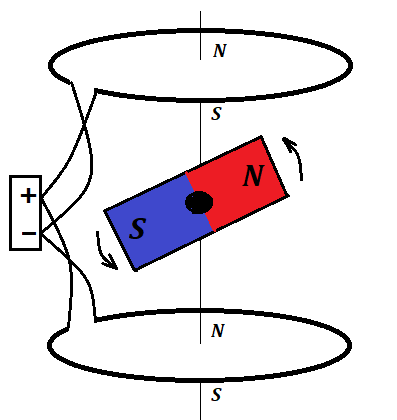
If at the moment our permanent magnet aligns with magnetic field of both
wire loops we change the direction of electric current in the loops to
an opposite, thus changing the polarity of the magnetic field they
generate, the permanent magnet in between will have to continue the
rotation until it will align again. Repeating this cycle creates the
rotation of a permanent magnet.
This rotation will not be smooth. The force of magnetic field attraction
will create a stronger moment of rotation when the direction of the
permanent magnet is perpendicular to a direction of the magnetic field
of the loops. This can be improved by certain additional details
described below.
The changing of the direction of the current in the wire loops now is
much easier than in the previous design because the wires are not
moving. Simple electronic switch working off some kind of a marker on a
rotating permanent magnet can signal its position and trigger the switch
of direction of the current.
Basically, the idea is finished here. But some very important improvements should be mentioned.
1. Instead of a single wire loop we can use a copper coil around an iron
core to make the magnetic field of this electromagnet stronger.
2. Instead of a pair of such electromagnets with switching the direction
after the permanent magnet in the middle turns by 180°, we can use two
pairs and properly engage another pair after 90° turn, switching off the
previous pair, thus creating a rotating magnetic field that results in a
smoother movement of a permanent magnet rotor. Even better, we can use
three pairs of electromagnets and engage another pair after each 60°,
switching off the previous two pairs. This will allow even smoother
rotation. In some DC motors they use even six pairs of electromagnets,
which results in a very uniform rotation with practically constant
angular speed.
The electronic switches that engage and disengage the coils can be designed for any type of coil arrangement.
3. Instead of a bar permanent magnet inside the circle of electromagnets
we can use a ring permanent magnet outside it. It creates a better
response to a revolving magnetic field of a stator, the rotation will be
smoother because of inertia of a rotating ring.
With all the above improvements the DC motor used in hard disk of computers and other devices looks like the one below
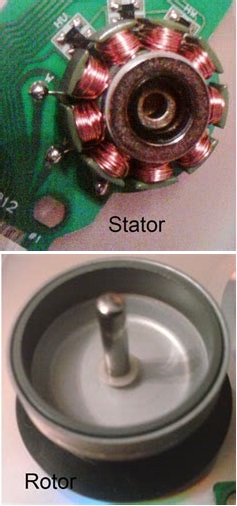
This DC motor has 9 electromagnets sequentially engaged after each 40° turn of a rotor.
Rotor is a ring-shaped permanent magnet that rotates around the electromagnets.
A marker on the rotor sends a signal to an electronic switch to indicate
the position of a rotor, which is used by electronics on the attached
board to properly engage the electromagnets of a stator.
The most important detail of this design of a DC motor is the rotating
magnetic field achieved through purely electronic means without any
moving parts. This results in a smooth rotation of a rotor made of a
ring permanent magnet around a stator.
No comments:
Post a Comment