Angle Refraction of Light
Let's analyze what happens with a flat wave front of light, when its parallel rays fall at an angle onto a border between two transparent substances with incident rays coming from a substance with a smaller refraction index (and, therefore, higher speed of light in this substance, since refraction index is a ratio of the speed of light in the vacuum to a speed of light in the substance). For example, flat wave front of light from air falls on a glass surface.
As we know from the previous lectures, there is a dependency between refraction indices n1, n2 of (or speeds of light V1, V2 in) two bordering substances and angles θ1 of incidence and θ2 of refraction of a ray of light falling on the border:
sin(θ1)/V1 = sin(θ2)/V2
or sin(θ1)·n1 = sin(θ2)·n2
This was derived from the Fermat's Principle of the Least Time.
Now we will use the Huygens principle to analyze this process from the wave theory viewpoint and demonstrate the same result.
Let's recall a simple illustration to Huygens Principle
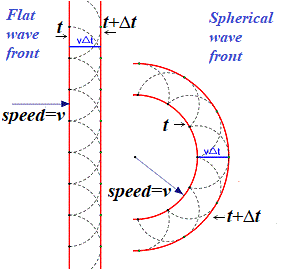
The picture above shows how a wave front propagates through space by assuming that each point of this wave front at time t is a source of oscillations propagated in all directions, reaching during the next interval of time Δt a surface of a small sphere of radius r=c·Δt around this point, where c is the speed of light. The resulting new wave front at time t+Δt will be a surface enveloping all these small spheres, that is tangent to each and every one of them.
Situation becomes more complex, when the speed of light is not the same at different points of the wave front because the wave front falls onto a different transparent substance, for example, it falls from the air onto a glass surface.
If, for example, a flat wave front falls from the air perpendicularly to a flat glass surface, the speed of light at different points of a wave front at the same time is the same, faster for wave front in the air and slower in the glass. The light will propagate in the same direction in the glass, as it was in the air.
But, if the flat wave front falls from the air at some acute incident angle onto flat glass surface or a spherical wave front falls on a flat glass surface, those point of the wave front that reached the glass earlier will emit light at a slower speed, so the propagation of the wave front will not be the same as on the picture above.
Let's examine the behavior of the flat wave front falling from the air onto a flat glass surface at an incident angle θ1 and analyze the shape and direction of propagation of the wave front at moment in time t+Δt, knowing its position and direction at time t.
The picture below represents a section of a set of synchronous parallel rays of light falling onto a flat air/glass border at an acute incident angle θ1.
(for a clearer view click the right mouse button on the picture and open it in another browser tab)
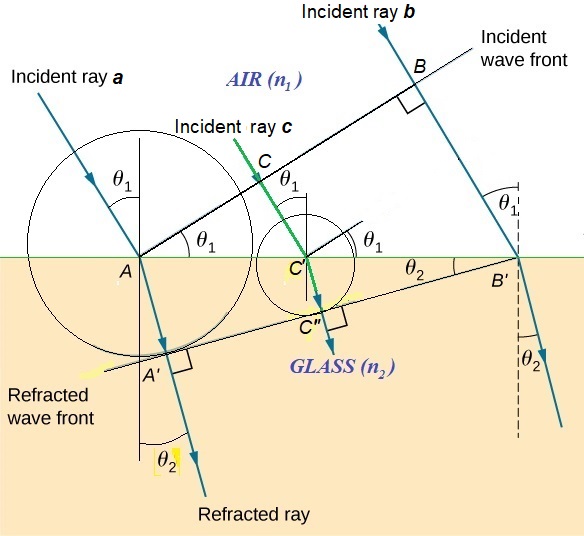
The wave front of light, consisting of parallel rays synchronously emitted by flat plane source, can be obtained by connecting points on different rays, where light comes at the same time. As the rays of this light come from some flat source and move in the uniform environment (air), the wave front will always be a plane perpendicular to rays.
There are three rays presented on the above picture out of the whole set of rays - those going through points A, C and B. We will call these rays a, c and b to correspond to points they pass.
Let's assume that at time t the wave front goes through these points A, C and B perpendicularly to the propagation in the air.
Because the incident angle of all those rays is not zero, different parallel among themselves rays will reach the border between air and glass at different time.
The first ray that touches the air/glass border at point A is ray a. Representing as ta the touch time for this ray, we can say
ta = t.
Next is an intermediate ray c that at time t goes through point C and later on at time tc touches the border at point C'.
Finally, ray b at time t goes through point B and later on (later than ray c) at time tb, which is greater than tc, touches the border at point B'.
Let's examine the wave front at time t+Δt, where Δt is the time difference between moments tb (the last ray to touch the glass surface) and ta (the first ray touching the glass surface).
All this period of time Δt=tb−ta ray a moved inside the glass with lower speed V2.
We don't know its direction, but can build a sphere around last known location (point A) at time ta of radius
ra=AA'=V2·(tb−ta)
centered at A.
The new wave front at time t+Δt will be tangent to this sphere.
Ray c moved in the air to point C' with higher speed V1 during time from ta to tc, which is a part of the Δt=tb−ta period, and the rest of the time from tc to tb moves inside the glass with lower speed V2.
So, we can build a sphere of radius
rc=C'C"=V2·(tb−tc)
centered at C', which the new wave front at time t+Δt should be a tangent to.
Ray b during the entire period of time Δt=tb−ta moved in the air with higher speed V1 along a known trajectory from point B to point B'.
The new wave front at time t+Δt should go through its end point B'.
First, let's find the interval of time Δt=tb−ta for ray b to travel from point B to touch the border at point B' or for ray a to travel from point A on the border into the glass to point A' (which we don't know) or for ray c to travel from point C in the air to, first, point C' on the border and then to C" in the glass (which we don't know).
Let the distance between the earliest to touch the border ray a and the latest b (that is, the length of AB) be d. Then the length BB' will be d·tan(θ1).
From this, taking into account the speed of light in the air V1,
Δt = tb−ta = d·tan(θ1)/V1
During the time from ta, when ray a crossed the border, to time tb, when ray b touched the border, ray a moves inside the glass to point A' with slower speed V2, while ray B moves in the air from point B to B' with higher speed V1.
By the time ray B reaches the border surface at point B' ray A will move inside the glass by a distance AA' that is shorter than BB' because it's speed in the glass is slower than that of ray B in the air.
Therefore,
ra = AA' = V2·(tb − ta) =
= V2·d·tan(θ1)/V1
During the same time ray c will partially travel through air with speed V1 and partially through glass with slower speed V2, which will bring it to a distance C'C" from the border inside the glass.
Assuming the distance between parallel rays b and c is x, the ray c will travel the time
τc1=(d−x)·tan(θ1)/V1
in the air.
The remaining time τc2=Δt−τc1 it will travel through glass with a lower speed V2, which will bring it on the distance
rc = C'C"=V2·τc2
from point on the border C'.
That gives
rc = V2·τc2 = V2·(Δt−τc1) =
= V2·x·tan(θ1)/V1
Since initially rays a, c and b were parallel to each other and they sustain the same refraction on the border between air and glass, the refracted rays will be parallel as well.
Consider now positions of our three rays at time t+Δt.
Ray a will go inside the glass from point A by a distance
ra = AA' = V2·d·tan(θ1)/V1
Ray c will go inside the glass from point C' by a distance
rc = C'C" = V2·x·tan(θ1)/V1
Ray b will be on the border at point B', that is will go inside the glass by a distance zero.
As we see, the distance inside the glass is changing from its maximum for ray a to zero at ray b. Considering variable x as changing from zero (when ray c coincides with ray b) to d (when ray c coincides with ray a), we see that the distance of penetration inside the glass, as a function of x, is linear.
Let's apply the Huygens Principle.
What follows from this is that, if we will make spheres around points of touching the glass for each ray, the radii of these spheres will linearly change from maximum for ray a to zero for ray b. Therefore, a surface that envelopes all these spheres will be a flat plane, which in a section presented on the picture above will be represented by a line A'B'.
From the above follows:
sin(θ2) = AA'/AB' =
= AA'·cos(θ1)/d =
= V2·d·tan(θ1)·cos(θ1)/(d·V1) =
= V2·sin(θ1)/V1
Therefore,
V1/sin(θ1) = V2/sin(θ2)
or
sin(θ1)/sin(θ2) = V1/V2
If we use refraction indices
ni = c/Vi, where c is the speed of light in the vacuum, the above formula is equivalent to
n1·sin(θ1) = n2·sin(θ2)
or
sin(θ1)/sin(θ2) = n2/n1
As you see, these formulae are identical to those derived before using Fermat's Principle of the Least Time.
No comments:
Post a Comment